- CBA-Material Description
- CBA-Asphalt Concrete
- CBA-Granular Base
- CBA-Stabilized Base
- CBA-Embankment/Structure Fill
Coal Bottom Ash/Boiler Slag - Material Description
Origin
Coal bottom ash and boiler slag are coarse, granular, incombustible materials that are collected from the bottom of furnaces that burn coal. The majority of coal bottom ash and boiler slag are produced at coal-fired electric utility generation stations, although bottom ash and boiler slag are produced by industrial and institutional coal-fired boilers and also from independent coal-burning electric generation facilities. The type of bottom ash or boiler slag produced depends on the type of furnace.Bottom Ash
The most common type of coal-burning furnace in the electric utility industry is the dry bottom pulverized coal boiler. When pulverized coal is burned in a dry bottom boiler, about 80 percent of the unburned material or ash is entrained in the flue gas and is captured and recovered as fly ash. The remaining 20 percent of the unburned material is dry bottom ash, a porous, glassy, dark gray material with a grain size similar to that of sand or gravelly sand.(1) Although similar to natural fine aggregate, bottom ash is lighter and more brittle and has a greater resemblance to cement clinker.(2) Bottom ash is collected at the bottom of the combustion chamber in a water-filled hopper and is removed by means of high-pressure water jets and conveyed by sluiceways to a decanting basin for dewatering followed by stockpiling and possibly crushing(1) According to the American Coal Ash Association (ACAA), during 2006, the U.S. utility industry generated 16.9 million metric tons (18.6 million tons) of bottom ash.(3)
Boiler Slag
There are two types of wet-bottom boilers that produce boiler slag: slag-tap and cyclone. The slag-tap boiler burns pulverized coal while the cyclone boiler burns crushed coal. Wet-bottom boiler slag is a term that describes the molten condition of the ash being drawn from the bottom of the furnaces. Both boiler types have a solid base with an orifice that can be opened to permit molten ash to flow into a hopper below. The hopper in wet-bottom furnaces contains quenching water. When the molten slag comes in contact with the quenching water, the ash fractures instantly, crystallizes, and forms pellets. High-pressure water jets wash the boiler slag from the hopper into a sluiceway which then conveys the ash to collection basins for dewatering, possible crushing or screening, and stockpiling.(4) The resulting boiler slag, often referred to as "black beauty", is a coarse, angular, glassy, black material.
When pulverized coal is burned in a slag-tap furnace, as much as 50 percent of the ash is retained in the furnace as boiler slag. In a cyclone furnace, which burns crushed coal, 70 to 85 percent of the ash is retained as boiler slag.(5) The ACAA estimates that during 2006, the utility industry in the United States generated 1.8 million metric tons (2.0 million tons) of boiler slag.(3)
A general diagram depicting the coal combustion steam generation process and the ash collecting points is presented in Figure 1. As shown in Figure 1, bottom ash or boiler slag is collected directly from the boiler/furnace and requires no separate system to collect the ash.
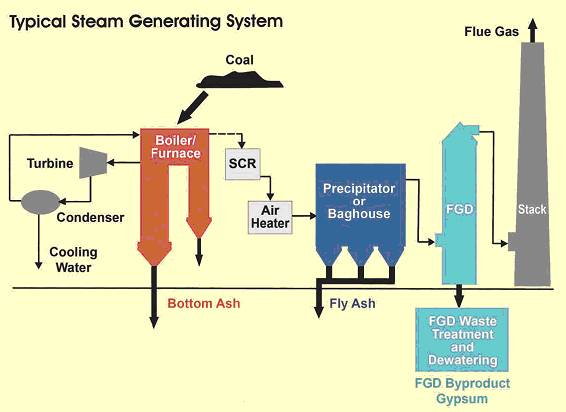
Figure 1. Production of coal combustion byproducts. (5)
Additional information on the use of bottom ash and boiler slag can be obtained from:
American Coal Ash Association (ACAA)
15200 E. Girard Ave., Ste. 3050
Aurora, Colorado 80014-3955
http://www.acaa-usa.org/
Coal Combustion Products Partnership (C2P2)
Office of Solid Waste (5305P)
1200 Pennsylvania Avenue, NW
Washington, DC 20460
http://www.epa.gov/epaoswer/osw/conserve/c2p2/index.asp
Electric Power Research Institute (EPRI)
3412 Hillview Road
Palo Alto, California 94304
http://my.epri.com
Edison Electric Institute (EEI)
1701 Pennsylvania Avenue, N.W.
Washington, D.C. 20004-2696
http://www.eei.org/
Green Highways Partnership
http://www.greenhighways.org/index.cfm
AASHTO Center for Environmental Excellence
444 North Capitol Street, NW Suite 249
Washington, D.C., 20001
202-624-5800
http://environment.transportation.org/
CURRENT MANAGEMENT OPTIONS
Beneficial useAccording to 2006 statistics on bottom ash usage, just over 45 percent of all bottom ash produced is used, mainly in transportation applications such as structural fill, road base material, and as snow and ice control products.(3) Bottom ash is also used as aggregate in lightweight concrete masonry units(6) and raw feed material for the production of Portland cement.(3;7;8) As the acceptance of the use of bottom ash increases, these markets have the potential to utilize all of the bottom ash produced annually in the U.S.(5) Figure 2 illustrates the common applications of coal bottom ash.
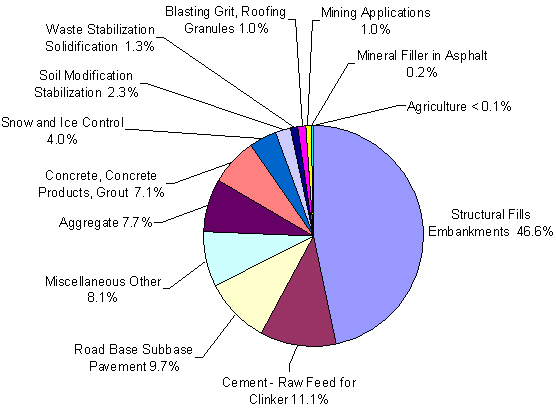
Figure 2. Bottom ash applications as a percentage of total reused. (3)
Nearly 84 percent of all boiler slag generated annually in the U.S. is utilized.(3) As shown in Figure 3, the leading boiler slag application is blasting grit and roofing shingle granules. Boiler slag is used in transportation applications including structural fills, mineral filler, and snow and ice control.(3) Boiler slag has also been used as aggregate in asphalt paving and as a road base and subbase.(3) Much of the boiler slag currently produced is from cyclone boilers that are falling out of favor due to high NOX emissions. As older cyclone boilers are retired, the amount of available boiler slag will decrease. To quantify this decline, 2.57 million tons of boiler slag were produced in 1996(5)compared to 2.03 million in 2006.(3)
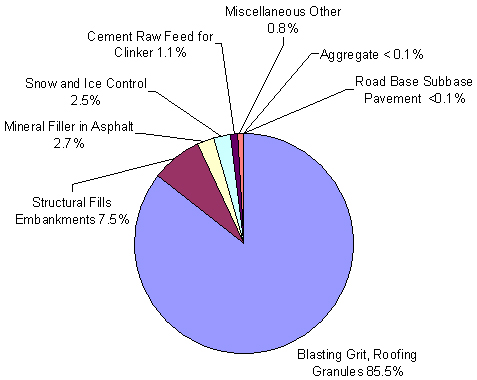
Boiler slag applications as a percentage of total reused. (3)
Regulatory guidelines for recycling of coal combustion byproducts have developed and evolved over the past few decades. The US EPA conducted two regulatory determinations on the management and use of coal combustion byproducts in 1993 and in 2000. Upon conducting these assessments, the EPA did not identify environmental hazards associated with the beneficial use of coal combustion products and concluded in both determinations that these materials did not warrant regulation as hazardous wastes.(9) In May 2000, the EPA made the statement, "we do not wish to place any unnecessary barriers on the beneficial use of fossil fuel combustion wastes so that they can be used in applications that conserve natural resources and reduce disposal costs."(9) This includes the beneficial use of coal combustion products in both encapsulated and unencapsulated transportation applications. The EPA recognizes that unencapsulated uses of coal combustion byproducts require proper hydrogeologic evaluation to ensure adequate groundwater protection.(10)
Disposal
Discarded bottom ash and boiler slag are either landfilled or sluiced to storage lagoons. When sluiced to storage lagoons, the bottom ash or boiler slag is usually combined with fly ash. This blended fly ash and bottom ash or boiler slag are referred to as ponded ash. Ponded ash is useable, but the engineering properties and behavior of ponded ash may vary and be similar to either fly ash or bottom ash-boiler slag, depending on the ratio of each in the ponded ash.(11) Because of differences in the density and particle size of fly ash and bottom ash-boiler slag, the coarser bottom ash-boiler slag particles settle first and the finer fly ash remains in suspension longer, thus producing a segregated layered deposit. Ponded ash can be reclaimed and stockpiled, during which time the ponded ash is dewatered. Under favorable drying conditions, ponded ash may be dewatered into a range of moisture that is near optimum moisture content. The higher the percentage of bottom ash-boiler slag, the easier ponded ash is to dewater. Reclaimed ponded ash has been used in stabilized base or subbase mixes and in embankment construction and can also be used as fine aggregate or filler material in flowable fill.
State Regulations
The U.S. Environmental Protection Agency (EPA) has delegated responsibility to the states to ensure that coal combustion by-products are properly used. Each state, therefore, should have specifications and environmental regulations. A map from the National Energy Technology Laboratory that links to a database of state regulations on the utilization and disposal of coal combustion by-products can be found here.
The state regulations database contains summary information on current regulations in each state and contact information for individuals with regulatory responsibility. A site maintained by the U.S. Federal Highway Administration (FHWA) contains a searchable library for all highway specifications across the country. This can be found here.
MARKET SOURCES
Although coal-burning electric utility companies produce ash, most utilities make use of commercial ash vendors to sell ash. In addition to commercial ash marketing firms, some coal-burning electric utility companies have formal ash marketing programs. Most coal-burning electric utility companies currently employ an ash management specialist whose responsibility is to monitor ash generation, quality, use, or disposal, and to interface with ash marketers. To identify an ash source, contact the local utility company or visit the American Coal Ash Association's web site at the like provided above.The market value of coal combustion byproducts is determined by several factors. These include the uses, dictated by the ash properties, distance to a user, and the state and local regulations that determine which ash use are allowed. An estimate of bottom ash cost for snow and ice control is between $3-6 per ton, while bottom ash used for road base costs approximately $4-8 per ton.(5)
HIGHWAY USES AND PROCESSING REQUIREMENTS
Asphalt Concrete Aggregate (Bottom Ash and Boiler Slag)Both bottom ash and boiler slag have been used as fine aggregate substitute in hot mix asphalt wearing surfaces and base courses, and in emulsified asphalt cold mix wearing surfaces and base courses. Bottom ash is more commonly used in base courses than wearing surfaces, although recent field and laboratory research has shown that hot mix asphalt with up to 15 percent bottom ash had comparable performance to control mixes.(12) Because of the hard durable particles, boiler slag has been used in wearing surfaces, base courses, and asphalt surface treatment or seal coat applications.
Screening of oversized particles and blending with other aggregates will typically be required to use bottom ash and boiler slag in paving applications. Pyrites that may be present in the bottom ash should also be removed prior to use. Pyrites (iron sulfide) are volumetrically unstable, expansive, and produce a reddish stain when exposed to water over an extended time period. Technologies exist for processing bottom ash that can provide a cost-effective method to remove impurities (i.e. unburnt coal and pyrite) so that bottom ash meets product quality targets.(13)
Granular Base
Both bottom ash and boiler slag have occasionally been used as unbound aggregate or granular base material for pavement construction. Bottom ash and boiler slag are considered fine aggregates in this use. To meet required specifications, the bottom ash or slag may need to be blended with other natural aggregates prior being used as a base or subbase material. Screening or grinding may also be necessary particularly for the bottom ash, where large particle sizes greater than 19 mm (3/4 in) are present in the ash.
Stabilized Base Aggregate
Bottom ash and boiler slag have been used in stabilized base applications. Stabilized base or subbase mixtures contain a blend of aggregates and cementitious materials that bind the aggregates to increase bearing strength. Types of cementitious materials typically used include Portland cement, cement kiln dust, or pozzolans with activators, such as lime, cement kiln dusts, and lime kiln dusts. These cementitous properties have been found in both coal bottom ash and boiler slag which make them attractive options for stabilized base. The pozzolanic or cement-like activity of these materials, which contributes to the time-dependent change in mechanical properties, can be controlled by adjusting the particle size through grinding. It should be kept in mind, however, that grinding is an expensive and time consuming process.(14) When constructing a stabilized base using either bottom ash or boiler slag, both moisture control and proper particle gradation are required. Deleterious materials such as pyrites should be removed.
Embankment or Backfill Material
Bottom ash and ponded ash have been used as structural fill materials for the construction of highway embankments and/or the backfilling of abutments, retaining walls, or trenches. These materials may also be used as pipe bedding in lieu of sand or pea gravel. To be suitable for these applications, the bottom ash or ponded ash should be near the optimum moisture content and free of pyrites and/or popcorn-like particles. For use as trench fill around pipes the ash should be non-corrosive. Reclaimed ponded ash must be adequately dewatered prior to use. Bottom ash may require screening or grinding to remove or reduce oversize materials, greater than 19 mm (3/4 in) in size.
Flowable Fill Aggregate
Bottom ash has been used as an aggregate material in flowable fill mixes. Ponded ash can also be used in flowable fill. Since most flowable fill mixes require low compressive strength to allow for future excavation, no processing of bottom ash or ponded ash is typically needed. Neither bottom ash nor ponded ash needs to be at any particular moisture content to be used in flowable fill mixes because the amount of water in the mix can be adjusted in order to provide the desired flowability.
MATERIAL PROPERTIES
Physical PropertiesBottom ashes have angular particles with very porous surface textures. The ash particles range in size from a fine gravel to a fine sand with very low percentages of silt-clay sized particles. Bottom ash is predominantly sand-sized, usually with 50 to 90 percent passing a 4.75 mm (No. 4) sieve and 0 to 10 percent passing a 0.075 mm (No. 200) sieve. The largest bottom ash particle sizes typically rang from 19 mm (3/4 in) to 38.1 mm (1½ in). Bottom ash is usually a well-graded material although variations in particle size distribution may be encountered in ash from the same power plant. Figure 4 compares the grain size distribution curves of bottom ash samples from several sources.

Figure 4. Grain size distribution curves of several bottom ash samples.(15;16;17;4)
Boiler slag has a smooth surface texture unless gases are trapped in the slag when quenched, which produces a vesicular or porous particle. Boiler slag from the burning of lignite or subbituminous coal tends to be more porous than from burning eastern bituminous coals.(18) Boiler slag is essentially a coarse to medium sand with 90 to 100 percent passing a 4.75 mm (No. 4) sieve and 5 percent or less passing a 0.075 mm (No. 200) sieve.(4) The grain size distribution curves of several boiler slag samples are shown in Figure 5.
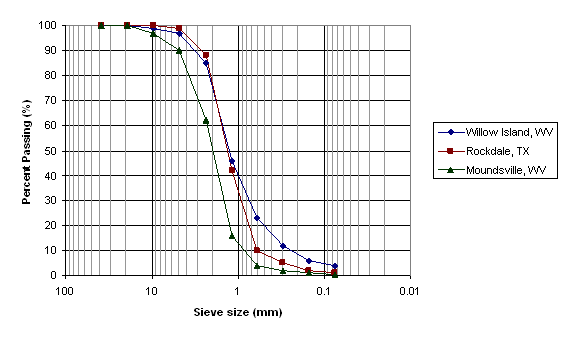
Figure 5. Grain size distribution curves of several boiler slag samples.(4)
The specific gravity of the dry bottom ash is a function of chemical composition, with higher carbon content resulting in lower specific gravity. Bottom ash with a low specific gravity has a porous or vesicular texture, a characteristic "popcorn particle" that readily degrade under loading or compaction.(19) Table 1 lists physical properties of bottom ash and boiler slag.
Table 1. Typical physical properties of bottom ash and boiler slag.
Property | Bottom Ash | Boiler Slag |
Specific Gravity (18) | 2.1 -2.7 | 2.3 - 2.9 |
Dry Unit Weight (18) | 7.07 - 15.72 kN/m3 (45 - 100 lb/ft3) | 7.43 - 14.15 kN/m3 (60 - 90 lb/ft3) |
Plasticity (18) | None | None |
Absorption (4) | 0.8 - 2.0% | 0.3 - 1.1% |
The physical properties of coal bottom ash and boiler slag vary depending on the type, source, and fineness of the parent fuel, as well as the operating conditions of the power plant.(14)
Chemical Properties
The chemical composition of bottom ash and boiler slag particles is controlled by the source of the coal and not by the type of furnace. Coal ash is composed primarily of silica (SiO2), ferric oxide (Fe2O3), and alumina (Al2O3), with smaller quantities of calcium oxide (CaO), potassium oxide (K2O), sodium oxide (Na2O), magnesium oxide (MgO), titanium oxide (TiO2), phosphorous pentoxide (P2O5), and sulfur trioxide (SO3). In bituminous coal ash, the three major components (SiO2, Fe2O3, and Al2O3) account for about 90 percent of the total components, whereas lignite and subbituminous coal ashes have relatively high percentages of CaO, MgO, and SO3.(20) Figures 6 and 7 present a chemical analysis of selected samples of bottom ash and boiler slag.

Figure 6. Chemical composition of selected bottom ash samples in percentage of total weight. (21;20;17;4;14)
Figure 7. Chemical composition of selected boiler slag samples in percentage of total weight.(4;14)
Sulfate, not shown in either Figures 6 or 7, is usually very low (less than 1.0 percent), unless pyrites are present in bottom ash or boiler slag.
Due to salt content and low pH, bottom ash and boiler slag may potentially be corrosive. When using bottom ash or boiler slag in an embankment, backfill, subbase, or even in a base course, the ash may come in contact with metal structures and cause corrosion. Therefore, evaluation of the corrosive nature of the bottom ash or boiler slag being used should be investigated. Corrosivity indicator tests normally used to evaluate bottom ash or boiler slag are pH, electrical resistivity, soluble chloride content, and soluble sulfate content. Materials are judged to be noncorrosive if the pH exceeds 5.5, the electrical resistivity is greater than 1500 ohm-centimeters, the soluble chloride content is less than 200 parts per million (ppm), or the soluble sulfate content is less than 1000 parts per million (ppm).(22)
Although similar in size and behavior to natural sand, the chemical composition of some bottom ash provides unique pozzolanic properties that, as with cementitious materials, can result in a favorable time-dependent increase in strength.(17) The strength increase generally occurs within the first seven days of curing. The pozzolanic activity of coal bottom ash can be improved by grinding.(14)
Mechanical Properties
Typical mechanical properties of bottom ash and boiler slag are listed in Table 2 including: compaction characteristics, durability, shear strength, bearing strength, resilient modulus, and hydraulic conductivity.
Table 2. Typical mechanical properties of bottom ash and boiler slag.
Property | Bottom Ash | Boiler Slag |
Maximum Dry Density kN/m3 (lb/ft3 ) (19;23) | 11.79 - 15.72 (75 - 100) | 12.89 - 16.04 (82 - 102) |
Optimum Moisture Content, % (19;23) | Usually <20 12 - 24 range | 8 - 20 |
Los Angeles Abrasion Loss % (4;24) | 30 - 50 | 24 - 48 |
Sodium Sulfate Soundness Loss % (4;24) | 1.5 - 10 | 1 - 9 |
Internal Friction Angle (drained) (18) | 38 - 42° 32 - 45° (<9.5 mm size) | 38 - 42° 36 - 46° (<9.5 mm size) |
California Bearing Ratio (CBR) % (25;2) | 21 - 110 | 40 - 70 |
Resilient Modulus (MR) regression coefficients (26;25) | K1 = 5 - 12 MPa | |
K2 = 0.52 | ||
Hydraulic Conductivity cm/sec (27;28) | 1 - 10-3 | 10-1 - 10-3 |
DESIGN CONSIDERATIONS
Although specifications for bottom ash and boiler slag use depend largely on the application, general suggestions can be made for improved use of bottom ash or boiler slag in roadway applications. The maximum dry density values of bottom ash and boiler slag are usually from 10 to 25 percent lower than that of naturally occurring granular materials. The optimum moisture content values of bottom ash and boiler slag are both higher than those of naturally occurring granular materials, with bottom ash being considerably higher in optimum moisture content than boiler slag. Moreover, the compaction curves of bottom ash generally have a flat shape, indicating that the material is insensitive to water content variations.(2;29)Boiler slag usually exhibits less abrasion loss and soundness loss than bottom ash because of the glassy surface texture and lower porosity.(30) Coal pyrites or soluble sulfate that are sometimes in bottom ash or boiler slag, may account for the sodium sulfate soundness loss values.(30) Reported friction angle values are within the same range as those for well-graded angular sand and are higher that Ottawa sand.(19;24) The internal friction angle of both bottom ash and boiler slag enables these materials to be used in properly designed slopes.
Compacted bottom ash used as a working platform and subsequently as a contributing subbase member in flexible pavement design has been studied.(26;23;25) California Bearing Ratio percentages as well as regression coefficients for the power function model to calculate resilient modulus, MR, are shown in Table 2. California Bearing Ratio values are comparable to those of high-quality gravel base materials. Laboratory and case study results show that with proper design and construction, compacted bottom ash provides adequate support as a working platform or subbase material.(26;25) Design charts for selecting the equivalent thickness of compacted bottom ash for working platforms are provided in reference 23, where a methodology for including the structural contribution of working platforms made from bottom ash or other alternative material is presented in 25.
Bottom ash and boiler slag can both be expected to have hydraulic conductivities that are within approximately the same range.(18) The hydraulic conductivity of bottom ash can be as low as 10-3 cm/s which is comparable to the lower limit obtained for natural materials with similar grain size distribution. Bottom ash or boiler slag are not typically susceptible to either liquefaction or frost heave. However, some of the finer ash materials may require the same consideration for design as fine grained fills.(11)
ENVIRONMENTAL CONSIDERATIONS
The major chemical components of bottom ash are the same as fly ash; however, due to the larger particle size and lower specific surface, bottom ash has a lower potential to leach trace elements than fly ash when used in the same application.(31) Bottom ash is typically used in bulk, unencapsulated applications such as in embankments, structural fills, and granular bases. Therefore, the dilution, fixation, and adsorption of trace elements that would occur if bottom ash were mixed with native soils is not expected.(26) Concentrations of trace elements from the leachate collected from bottom ash test sections have been shown to be higher than those collected from control sections as well as those collected from fly ash test sections.(26) The possibility of groundwater contamination by trace elements that are commonly associated with coal combustion by-products is a concern. Unencapsulated bottom ash or boiler slag use requires good management to ensure the environment is not impacted negatively. In particular, areas with sandy soils possessing high hydraulic conductivities and areas near shallow groundwater or drinking aquifers should be given careful consideration. An evaluation of groundwater conditions, applicable state test procedures, water quality standards, and proper construction are all necessary considerations in ensuring a safe final product.(10)Leachate studies conducted according to methods outlined in Table 3 would provide valuable information in gauging the environmental suitability of coal bottom ash and boiler slag.
Table 3. Extraction conditions for different standard leaching tests.(32)
Test Procedure | Method | Purpose | Leaching Medium | Liquid-Solid Ratio | Particle Size | Time of Extraction |
Water Leach Test | ASTM D3987-06 | To provide a rapid means of obtaining an aqueous extract | Deionized water | 20:1 | Particulate or monolith as received | 18 hr |
TCLP | EPA SW-846 Method 1311 | To compare toxicity data with regulatory level. RCRA requirement.< | Acetate buffer* | 20:1 | < 9.5 mm | 18 hr |
Extraction Procedure Toxicity (EP Tox) | EPA SW-846 Method 1310 | To evaluate leachate concentrations. RCRA requirement. | 0.04 M acetic acid (pH = 5.0) | 16:1 | < 9.5 mm | 24 hr |
Multiple Extraction Procedure | EPA SW-846 Method 1320 | To evaluate waste leaching under acid condition | Same as EP Toxicity, then at pH = 3.0 | 20:1 | < 9.5 mm | 24 hr extraction per stage |
Synthetic Precipitation Leaching Procedure (SPSL) | EPA SW-846 Method 1312 | For waste exposed to acid rain | DI water, pH adjusted to 4.2 to 5 | 20:1 | < 9.5 mm | 18 hr |
* Either an acetate buffered solution with pH = 5 or acetic acid with pH = 3.0 |
Aside from laboratory testing, lysimeter monitoring can provide field information on trace element release and leachate flow. A lysimeter is a device that collects water from overlying materials that can be tested for soluble constituents that were dissolved during rainwater percolation through the material.
A laboratory batch water leach test, column leach test, and below subbase lysimeter study evaluated leachate from bottom ash. Leachates were analyzed for concentrations of cadmium (Cd), chromium (Cr), selenium (Se), and silver (Ag) and compared to groundwater quality standards for Wisconsin. Peak concentrations in the lysimeters below 60 cm of bottom ash were all above peak concentrations found from the laboratory water leach test and were above the peak concentrations from the laboratory column leach test for Cd, Se, and Ag. Peak Cd and Se concentrations in the leachate from the field lysimeters exceeded the Wisconsin groundwater standard. However, with application of dilution factors to account for the reduction in concentration expected between the bottom of the pavement structure and the groundwater table, concentrations would not exceed the groundwater quality standards if the bottom ash layer is at least 1 m above the groundwater table.(33)
Modeling
Models currently used to simulate leaching from pavement systems and potential impacts to groundwater include STUWMPP,(34) IMPACT,(35) HYDRUS-2D,(36)(37)(38)WiscLEACH,(39) and IWEM.(40) Among these models STUWMPP, IMPACT, WiscLEACH and IWEM are in the public domain. STUWMPP employs dilution–attenuation factors obtained from the seasonal soil compartment (SESOIL) model to relate leaching concentrations from soils and byproducts to concentrations in underlying groundwater. IMPACT was specifically developed to assess environmental impacts from highway construction. Two dimensional flow and solute transport are simulated by solving the advection dispersion reaction equation using the finite difference method.(39).
WiscLEACH combines three analytical solutions to the advection-dispersion-reaction equation to assess impacts to groundwater caused by leaching of trace elements from CCPs used in highway subgrade, subbase and base layers. WiscLEACH employs a user friendly interface and readily available input data along with an analytical solution to produce conservative estimates of groundwater impact.(39)
The U.S. EPA's Industrial Waste Management Evaluation Model (IWEM), although developed to evaluate impacts from landfills and stock piles, can help in determining whether ash leachate will negatively affect groundwater. IWEM inputs include site geology/hydrogeology, initial leachate concentration, metal parameters, and regional climate data. Given a length of time, the program will produce a leachate concentration at a control point (such as a pump or drinking well) that is a known distance from the source. In addition, Monte Carlo simulations can provide worst-case scenarios for situations where a parameter is unknown or unclear. In comparing IWEM to field lysimeter information, IWEM over predicted the leachate concentrations and could be considered conservative. Overall, however, IWEM performed satisfactorily in predicting groundwater and solute flow at points downstream from a source.(41) A byproducts module for IWEM will be offered by the EPA in the near future.
A source for information on assessing risk and protecting groundwater is the EPA's "Guide for Industrial Waste Management" (42) which can be found at:http://www.epa.gov/industrialwaste/guide.asp
Finally, due to the variability in bottom ash and boiler slag composition between coal plants, industry-wide generalizations about the environmental impact of bottom ash and boiler slag cannot be made. Also, because of the variety of leachate testing methods and the variety of standards and regulations to compare these test results to, state regulations should be identified and followed when determining the environmental suitability of bottom ash or boiler slag from a particular source.
REFERENCES
A searchable version of the references used in this section is available here. A searchable bibliography of bottom ash and boiler slag literature is available here.- Steam, its generation and use. 39th ed. New York: Babcock & Wilcox; 1978.
- Rogbeck J, Knutz A. Coal bottom ash as light fill material in construction. Waste Management 1996;16(1-3):125-8.
- American Coal Ash Association (ACAA). 2006 coal combustion product (CCP) production and use. Aurora, CO: American Coal Ash Association; 2007.
- Moulton LK. Bottom ash and boiler slag. In: Proceedings of the third international ash utilization symposium. Washington, DC: U.S. Bureau of Mines; 1973.
- NETL National Energy Technology Laboratory. Clean coal technology: Coal utilization by-products. Washington, DC: Department of Energy Office of Fossil Energy; 2006 August. Topical report no. 24.
- ASTM C331-05 standard specification for lightweight aggregates for concrete masonry units. In: Annual book of ASTM standards. West Conshohocken, Pennsylvania: ASTM; 2005.
- Cheriaf M, Cavalcante Rocha J, Pérao J. Pozzolanic properties of pulverized coal combustion bottom ash. Cement and Concrete Research 1999;29(9):1387-91.
- Canpolat F, Yilmaz K, Köse MM, Sümer M, Yurdusev MA. Use of zeolite, coal bottom ash and fly ash as replacement materials in cement production. Cement and Concrete Research 2004 5;34(5):731-5.
- Vivek Tandon and Miguel Picornell. Safe disposal of fly ash in pavement or earth structures not requiring high strength materials. Proceedings of the Geo-Congress, Oct 18-21 1998, Boston, MA, USA: ASCE, Reston, VA; 1998.
- Environmental Protection Agency (EPA), Federal Highway Administration (FHWA). Using coal ash in highway construction - A guide to benefits and impacts. ; 2005. Report nr EPA-530-K-002:ID: 151.
- ASTM E2277-03 standard guide for design and construction of coal ash structural fills. In: Annual book of ASTM standards. West Conshohocken, Pennsylvania: American Society for Testing and Materials; 2003.
- Ksaibati K, Sayiri, S. R. K. Utilization of Wyoming bottom ash in asphalt mixes. Department of Civil & Architectural Engineering, University of Wyoming; 2006 March.
- Groppo J, Robl T. Construction fill sand production from bottom ash at Mill Creek Station. EPA; 2003 December. Case study No. 7.
- Özkan Ö, Yüksel I, Muratoglu Ö. Strength properties of concrete incorporating coal bottom ash and granulated blast furnace slag. Waste Management 2007;27(2):161-7.
- Katz A, Kovler K. Utilization of industrial by-products for the production of controlled low strength materials (CLSM). Waste Management 2004;24(5):501-12.
- Kim B, Prezzi M. Compaction characteristics and corrosivity of Indiana class-F fly and bottom ash mixtures. Construction and Building Materials; In Press, Corrected Proof.
- Kumar S, Vaddu P. Time dependent strength and stiffness of PCC bottom ash-bentonite mixtures. Soil and Sediment Contamination 2004;13(4):405-13.
- Majizadeh K, Bokowski G, El-Mitiny R. Material characteristics of power plant bottom ashes and their performance in bituminous mixtures: A laboratory investigation. In: Proceedings of the fifth international ash utilization symposium. Morgantown, West Virginia: Department of Energy; 1979.
- Lovell CW, Ke TC, Huang WH, Lovell JE. Bottom ash as highway material. In: 70th annual meeting of the transportation research board. Washington, DC: Transportation Research Board; 1991.
- Kim B, Prezzi M, Salgado R. Geotechnical properties of fly and bottom ash mixtures for use in highway embankments. Journal of Geotechnical and Geoenvironmental Engineering 2005;131(7):914-24.
- Andrade LB, Rocha JC, Cheriaf M. Evaluation of concrete incorporating bottom ash as a natural aggregates replacement. Waste Management; In Press 2007.
- Ke TC, Lovell CW. Corrosivity of Indiana bottom ash. Transportation Research Record 1992;1345:113-117.
- Tanyu BF, Benson CH, Edil TB, Kim W. Equivalency of crushed rock and three industrial by-products used for working platforms during pavement construction. Transportation Research Record 2004(1874):59-69.
- Huang WH. The use of bottom ash in highway embankment and pavement construction. Purdue University; 1990. p. 317.
- Tanyu BF, Kim W, Edil TB, Benson CH. Development of methodology to include structural contribution of alternative working platforms in pavement structure. Transportation Research Record 2005(1936):70-7.
- Edil TB, Benson CH, Bin-Shafique MS, Tanyu BF, Kim W, Senol A. Field evaluation of construction alternatives for roadways over soft subgrade. Transportation Research Record 2002;1786(1):36-48.
- Prakash K, Sridharan A. A geotechnical classification system for coal ashes. Proceedings of the Institution of Civil Engineers, Civil Engineering 2006 04;159(2):91-8.
- Siddiki NZ, Kim D, Salgado R. Use of recycled and waste materials in Indiana. Transportation Research Record 2004(1874):78-85.
- Tanyu BF, Kim W, Edil TB, Benson CH. Comparison of laboratory resilient modulus with back-calculated elastic moduli from large-scale model experiments and FWD tests on granular materials, In: G. Durham, A. Marr, W. De Groff, editors. Resilient modulus testing for pavement components. West Conshohocken, PA: ASTM; 2003.
- Moulton LK, Seals RK, Anderson DA. Utilization of ash from coal burning power plants in highway construction. Transportation Research Record 1973 (430):26-39.
- Ramme BW, Tharaniyil M. Coal combustion products utilization handbook. Milwaukee, WI: We Energies; 2004.
- Bin-Shafique MS, Benson CH, Edil TB. Geoenvironmental assessment of fly ash stabilized subbases. University of Wisconsin – Madison, Madison, WI: Geo Engineering, Department of Civil and Environmental Engineering; 2002 March 11, Geo Engineering Report No. 02-03.
- Sauer JJ, Benson CH, Edil TB. Metals leaching from highway test sections constructed with industrial byproducts. University of Wisconsin – Madison, Madison, WI: Geo Engineering, Department of Civil and Environmental Engineering; 2005 December 27, Geo Engineering Report No. 05-21.
- Friend M, Bloom P, Halbach T, Grosenheider K, Johnson M. Screening tool for using waste materials in paving projects (STUWMPP). Office of Research Services, Minnesota Dept. of Transportation, Minnesota; 2004. Report nr MN/RC–2005-03.
- Hesse TE, Quigley MM, Huber WC. User’s guide: IMPACT—A software program for assessing the environmental impact of road construction and repair materials on surface and ground water. NCHRP; 2000. Report nr NCHRP 25-09.
- Simunek J, Sejna M, van Genuchten, M. T. The HYDRUS-2D software package for simulating the two-dimensional movement of water, heat, and multiple solutes in variably-saturated media. Golden, Colorado: International Ground Water Modeling Center; 1999. Report nr IGWMC - TPS - 53.
- Bin-Shafique MS, Benson CH, Edil TB. Leaching of heavy metals from fly ash stabilized soils used in highway pavements. University of Wisconsin – Madison, Madison, WI: Geo Engineering, Department of Civil and Environmental Engineering; 2002. Report nr 02-14.
- Apul D, Gardner K, Eighmy T, Linder E, Frizzell T, Roberson R. Probabilistic modeling of one-dimensional water movement and leaching from highway embankments containing secondary materials. Environmental Engineering Science 2005;22(2):156–169.
- Li L, Benson CH, Edil TB, Hatipoglu B. Groundwater impacts from coal ash in highways. Waste and Management Resources 2006;159(WR4):151-63.
- Environmental Protection Agency (EPA). Industrial waste management evaluation model (IWEM) User’s guide. Washington, DC: US EPA; 2002. Report nr EPA530-R-02-013.
- Melton JS, Gardner KH, Hall G. Use of EPA’s industrial waste management evaluation model (IWEM) to support beneficial use determinations. U.S. EPA Office of Solid Waste and Emergency Response (OSWER); 2006.
- Guide for Industrial Waste Management [Internet]; c2006. Available from: http://www.epa.gov/epaoswer/non-hw/industd/guide/index.asp.
Coal Bottom Ash/Boiler Slag - Asphalt Concrete
INTRODUCTION
In the United States, about 3.7 million km (2.3 million miles) or 55 percent of all roadways are surfaced with asphalt concrete, making asphalt concrete a critical component of the nations infrastructure.(1;2) Asphalt pavements in new roadway construction typically requires a large amount of virgin materials. Bottom ash and boiler slag can be used as an aggregate replacement, providing a substantial savings to both highway agencies and utility companies. Bottom ash has been used as aggregates in hot mix asphalt (HMA) base courses, in emulsified asphalt cold mixes, and in shoulder construction. Boiler slag has been used in the same applications as bottom ash and also as wearing surfaces, emulsified asphalt cold mix bases or surfaces, and asphalt surface treatments or seal coats.Bottom ash produced in dry bottom boilers is usually sufficiently well-graded to meet gradation requirements for asphalt concrete. However, bottom ash particles are less durable than conventional aggregates. Consequently, bottom ash is better suited for use in base course and shoulder mixtures or in cold mix applications, as opposed to wearing surface mixtures. Most bottom ash paving applications have been cold mix projects on low-volume secondary roadways.
Boiler slag produced in wet bottom boilers is uniformly sized, and consists of hard, durable, glassy particles. Boiler slag is typically blended with other fine aggregates to meet gradation requirements of asphalt concrete, but because of the hard, durable particles and resistance to surface wear boiler slag is used more frequently in asphalt paving than bottom ash. Boiler slag enhances HMA wearing surfaces, because boiler slag has an affinity for asphalt and has a dust-free surface, which increases adhesion and anti-stripping characteristics. Boiler slag has also been used successfully as a seal-coat aggregate for bituminous surface treatments to enhance skid resistance.(3)
PERFORMANCE RECORD
Bottom ash and boiler slag have been used as fine aggregates in asphalt paving mixtures since the early 1970's. The American Coal Ash Association reported that, 40,800 metric tons (45,000 tons) of boiler slag and over 17,200 metric tons (19,000 tons) of bottom ash were used in asphalt paving during 2006.(4)Bottom Ash
Bottom ash may contain pyrites or lightweight, porous "popcorn" particles. As a result, bottom ash is not an ideal aggregate source in HMA paving mixes, especially wearing surfaces. Bottom ash has been used more frequently in cold mix emulsified asphalt mixtures, hot mix base course mixtures, and in shoulder construction, where durability considerations are not as critical as in hot mix wearing surface mixtures.(5)
The most extensive use of bottom ash in bituminous paving has been in West Virginia, where, during the 1970's and 1980's, bottom ash was cold mixed with 6 to 7 percent by weight of emulsified asphalt and used in the paving of secondary roads where durability concerns are reduced. Similar paving has also been done in eastern Ohio.(6) To improve the characteristics of a cold mix containing bottom ash, boiler slag can be included.
Because of the potential market for bottom ash as a partial replacement for aggregate in HMA, bottom ash continues to be researched and fielded tested in HMA. In West Virginia, there have been indications of problems with paving mixtures containing bottom ash in which pyrite contamination in the bottom ash had not been considered. Pyrite particles will weather in service, despite being coated with asphalt cement, causing popouts and deep red stains in the pavement surface.(7) Iron pyrites should be removed before bottom ash is used as an aggregate replacement material.(2)
Recent studies have indicated that bottom ash may possess desirable engineering properties and will not degrade HMA performance properties when used to replace a portion of the fine aggregate in an asphalt mix.(2) No more than 30 percent of bottom ash as aggregate replacement is recommended, mixes with 50 percent or more of bottom ash in asphalt pavements were found to have unacceptable stabilities(8). In a study where 15 percent bottom ash replaced aggregate, HMA mixes prepared with bottom ash did not show any significant degradation in performance properties when compared to control mixes. The use of bottom ash as 15 percent replacement of aggregate in HMA mixes maintained desirable strength properties, low temperature properties, and rutting properties. However, the addition of bottom ash required an increase in asphalt content.(1)
Boiler Slag
Boiler slag is used more frequently in HMA than bottom ash because of the hard, durable particles and resistance to surface wear. In addition, boiler slag has an affinity for asphalt and a dust-free surface that aids in asphalt adhesion and resistance to stripping.(3) Boiler slag has a black color that does not fade, which aids in the melting of snow from the road surface.(9) Since boiler slag is poorly-graded, blending with other aggregates is needed to meet specifications for use in asphalt mixtures. The recommended percentage of boiler slag should be less than 50 percent to maintain paving mixture stability.(7)
Boiler slag was first used in asphalt paving in Hammond, Indiana, where, on an experimental basis, bottom slag was blended with conventional aggregate to solve a problem of aggregate polishing. The success of that project and several other demonstration projects in Indiana led to the acceptance and use of boiler slag in Indiana and several other states, including Ohio, Michigan, Missouri, and West Virginia. Boiler slag has also been used as an aggregate in HMA paving in a number of cities such as Cincinnati and Columbus, Ohio, as well as in Tampa, Florida.(10)
Boiler slag has been used successfully as a seal coat aggregate for bituminous surface treatments. Boiler slag provides better coverage per mile than limestone chips and retains a rich black color that is an excellent contrast to road strip colors. The attributes of boiler slag often lead to specifying boiler slag for rehabilitation of airport runways and taxiways.(11)
MATERIAL PROCESSING REQUIREMENTS
CleaningPyrites, which are more prevalent is high sulfur coal, are typically removed from coal during pulverizing and/or cleaning operations prior to burning. Commingling the rejected pyrite with bottom ash is a practice at some power plants. Material handling operations should be modified to keep pyrite and bottom ash-boiler slag separate.(12) Pyrites (iron sulfide) are volumetrically unstable, expansive, and produce a reddish stain when exposed to water over an extended time period. If pyrites are present in the bottom ash or boiler slag, they should be removed by electromagnets, media separation, or other means. Technologies typically used for bottom ash processing can provide a cost-effective method to remove impurities (i.e. unburnt coal and pyrite) so that bottom ash meets product quality targets.(13)
Screening
Oversize or agglomerated popcorn particles may be present in some bottom ash sources and should be removed by screening the material with a 19 mm (¾ in) or 12.7 mm (½2 in) screen.
Blending
Boiler slag will almost always require blending with other aggregate sources to meet gradation specifications. Although more well-graded than boiler slag, bottom ash may require blending with aggregates.
Drying
Aggregates used to produce HMA are dried before blending with asphalt cement; therefore, moisture that may be present in bottom ash or boiler slag should be removed. Excessive moisture in the aggregates will reduce the production rate of paving material due to the additional drying time required. Both bottom ash and boiler slag are relatively easy to dewater, particularly boiler slag, which consists of glassy particles. Ponded ash, which is usually a mixture of fly ash and bottom ash or boiler slag, should be stockpiled and allowed to drain to a surface dry condition.
When used in a cold mix application, bottom ash should be at least surface dry so that moisture does not interfere with the coating of the ash particles by the emulsified asphalt. Boiler slag should also be in a surface dry condition when used as a seal coat aggregate.
ENGINEERING PROPERTIES
Properties of bottom ash and boiler slag that are of particular interest when used in asphalt concrete include gradation, specific gravity, absorption, and durability.Gradation: ASTM D1073 defines a fine aggregate in asphalt paving mixtures as an aggregate that passes the 9.5 mm (3/8 inch) sieve.(14) Boiler slag typically meets maximum size requirement for fine aggregate, while bottom ash sources may require minimal screening of oversize material to satisfy the definition of a fine aggregate. Because boiler slag is typically poorly-graded, blending with other aggregates may be needed to meet gradation requirements. Bottom ash is typically a well-graded sand-sized material.
Specific Gravity:Specific gravity is a good indicator of the quality of a material. The specific gravity of bottom ash and boiler slag depend on the mineralogical composition of the material as well as the porosity of the particles. A dry bottom ash with a high iron content may have a specific gravity as high as 3.4,(12) while a bottom ash with a large percentage of both porous and popcorn particles may be as low as 1.6.(2) In general, the lower the percentage of porous particles, the higher the specific gravity.
Absorption:Bottom ash generally has lower specific gravity and higher absorption values than limestone sand, while boiler slag is comparable in specific gravity with lower absorption than limestone sand.(15) The water absorption for bottom ash reported in the literature ranges from 0.3(15) to 6.10(16) percent. Due to the porous nature of bottom ash particles, the absorption of asphalt binder is higher than conventional fine aggregate. Hence, from a purely economic standpoint, bottom ash may not be a cost effective choice for asphalt aggregate.(17)
To reduces the asphalt consumption and improve friability of bottom ash particles, sulfur modification to coat bottom ash particles is being researched. Sulfur-modified bottom ash mixtures in which bottom ash represents 50 to 100 percent of the aggregate fraction compare favorably with typical surface course mixtures in terms of strength, durability, and asphalt demand.(18)
Durability: Bottom ash and boiler slag exhibit marginal durability as measured by the Los Angeles abrasion test, with bottom ash percent loss values between 30 and 50 and boiler slag somewhat lower. Most bottom ash samples have some friable particles, while boiler slag normally does not. Los Angeles Abrasion test results have shown that bottom ash samples are not as sound or durable as natural aggregate. However, the test results fall within the specifications of a maximum 50 percent loss by abrasion.
Soundness: Soundness values are generally found to be within ASTM C88(19) weight loss specifications of not more than 15 percent after five cycles when sodium sulfate is used, or not more than 20 percent after five cycles when magnesium sulfate is used.(6) Values for both boiler slag and bottom ash are generally found to be less than 10 percent.(15) Boiler slag has lower sulfate soundness loss because of the glassy surface texture and low porosity while bottom ash with porous popcorn particles has the highest loss.(12)
DESIGN CONSIDERATIONS
Mix DesignBottom Ash
Bottom ash is used less than boiler slag in asphalt paving because bottom ash has a higher asphalt absorption, some particles may be friability, and there is a potential for pyrites to be mixed into bottom ash from some sources. Most of the paving experience with bottom ash has been in cold mixes.
Because of the presence of friable popcorn particles in dry bottom ash that can break down under compaction, bottom ash is more appropriate as a base course rather than surface mixtures.(20) In addition, the porous particles in bottom ash absorb asphalt; therefore, asphalt contents of mixes containing bottom ash will be higher than mixes with conventional aggregates. Although the asphalt contents of mixes containing bottom ash will be greater than the asphalt contents of conventional asphalt paving mixes, the total weight of asphalt cement used should not be significantly greater because of the low unit weight of the bottom ash. Bottom ash mixes are also likely to have relatively high air void contents. The high air voids are attributable to the rough surface texture of bottom ash particles, which also produces a high angle of internal friction.(21)
Bottom ash may contain iron pyrites that reduce pavement strength. For this reason, no more than 30 percent of the aggregate in a asphalt pavement mix should be replaced with bottom ash.(2)
The comparatively high optimum asphalt content of mixtures using bottom ash as the only aggregate can be reduced by combining bottom ash with conventional aggregates. Research has shown that sulfur modified bottom ash mixes containing 50 to 100 percent bottom ash aggregate replacement can be achieved with 7.5 percent or less asphalt.(18)
Moisture-induced damage of asphalt pavement, referred to as stripping, reduces pavement life. Stripping occurs when moisture causes a loss of bond between aggregate and asphalt binder. Lime additives minimize the moisture susceptibility of pavement mixes.(2) The addition of lime in bottom ash mixtures also improves the indirect tensile strength and the tensile strength ratio.(20) Generally, 1 to 1.5 percent lime by dry weight of aggregate is used. Mixes containing large amounts of fine aggregate will require additional lime because of the increased surface area of the aggregate. Usually three forms of lime are used: hydrated lime (CaOH2), quick lime (CaO), and Dolomitic limes (both types S and N). Coating dry aggregate surfaces with dry hydrated lime may be difficult. Therefore, dry hydrated lime can be added to damp aggregate that contains 3 to 5 percent water content by weight of lime.(2)
Boiler Slag
The uniform gradation and smooth surface texture commonly associated with most boiler slags require that these materials be blended with other aggregates for use in asphalt paving mixtures. The blend proportions of boiler slag and conventional aggregates are designed to meet gradation specifications. Percentages of aggregate replaced by boiler slag range from 40 to 50 percent by weight of the total mix.(7) The type of aggregate used along with the relative proportions of boiler slag and aggregate influence the engineering properties of a pavement.(7)
Marshall stability and flow values decrease as the percentage of boiler slag increases for a given compactive effort. Mixes blended with rounded siliceous aggregates, such as uncrushed river sand, result in lower quality mixtures than blends containing crushed stone, which possess more desirable angularity and surface texture. Blending crushed stone aggregates with boiler slag is recommended because boiler slags lack microtexture that increases the aggregate-asphalt bond and to provide skid resistance.(15)
Optimum skid resistance using boiler slag is achieved in open graded sand mixes where boiler slag is the top-sized aggregate. However, such mixes should limit the percentage of boiler slag in the mix and avoid low filler content. Rounded river sands should also be avoided. Boiler slag does not appear to be as helpful in terms of skid resistance in coarse graded mixtures, especially if the coarse aggregate is polish susceptible.(7)
The laboratory effort and method of compaction effects pavement properties of mixes containing boiler slag. Kneading compaction improves the stability and flow characteristics compared to Marshall drop hammer compaction. Obtaining adequate compaction is essential with boiler slag mixtures. Optimum compaction is produced by blending boiler slag with well-graded, angular, rough-textured aggregate and limiting the percentage of boiler slag to 50 percent. Porous boiler slag can be used in greater percentages, but excessively porous slag are weak and can crush.(21) In addition, porous boiler slag may absorb more asphalt than typical boiler slag, thereby requiring a higher percentage of asphalt cement.
Boiler slag asphalt mixtures, evaluated using the Marshall immersion-compression test,(22) retain stability in the presence of water.(7)
Structural Design
Conventional AASHTO pavement structural design methods are appropriate for asphalt pavements incorporating bottom ash or boiler slag.(23)
Similarly, pavement thickness design procedures for cold mix overlays containing bottom ash or boiler slag are the same procedures used for cold mix overlays using conventional aggregates. Modified structural numbers (SN) for cold mix overlays containing bottom ash and/or boiler slag are the same as conventional cold mix overlays.
CONSTRUCTION PROCEDURES
Bottom Ash and Boiler SlagMaterial Handling and Storage
Bottom ash and boiler slag can be stored, stockpiled, and handled using the same methods and equipment as conventional aggregates. However, pyrites should be removed prior to using bottom ash in asphalt paving. Boiler slag mixtures with acceptable skid resistance that employ boiler slag as the top size aggregate can be designed by limiting the percentage of boiler slag in the mix. Boiler slag asphalt pavement mixtures should avoid open-graded mixtures with low filler content.
Mixing, Placing, and Compacting
The same methods and equipment used for mixing, placing, and compacting conventional pavements are applicable for asphalt pavements containing bottom ash or boiler slag. In hot mix applications, bottom ash or boiler slag are typically blended with other aggregates using conventional equipment. Dry bottom ash used in cold mix applications may not require blending and can be prepared by mixing with emulsified asphalt at a central pugmill mixing plant. Cold mix asphalt containing bottom ash or boiler slag can be prepared in advanced and stockpiled for 10 or more days.(8)
Cold mixes containing bottom ash can be placed with a paver, spreader box, or can be end dumped and leveled with a grader. Laydown characteristics of dry bottom ash cold mixes placed with either a spreader box or a conventional paving machine are the same as conventional mixes. Spreader box lifts of up to 200 mm (8 in) uncompacted mix can be placed. Lifts greater than 200 mm (8 in) in loose thickness may be difficult to compact.(8) Adequate compaction is usually achieved with several passes of a pneumatic roller followed by a steel-wheeled roller.(8)
ENVIRONMENTAL CONSIDERATIONS
Leaching of metals and air quality during construction are two environmental issues associated with using bottom ash or boiler slag in asphalt pavement. Bottom ash and boiler slag consist of the same chemical components as fly ash; therefore there exists the potential to leach trace elements. Because bottom ash and boiler slag have larger particles and less surface area per unit volume, the potential to leach trace elements is reduced. In addition, coal combustion products mixed in asphalt pavement is considered an encapsulated application that further reduces the potential to leach elements. A recent leachate study was conducted on test strips of asphalt concrete with bottom ash. Although trace elements were observed in the leachate, there was no evidence that the use of coal ash in asphalt pavements was the source.(24)Air quality during construction is and additional environmental concern involving the use of bottom ash as a highway material.(2) Material handling precautions should be taken to protect workers and the public from dusting during delivery and construction.(25)
UNRESOLVED ISSUES
Bottom ash that contains friable particles should be avoided in asphalt surface mixes unless precrushed before being mixed with asphalt. The performance of wearing surface mixes with precrushed bottom ash aggregate should be evaluated in comparison with more conventional asphalt paving mixes. Additional research into modifying bottom ash, i.e. sulfur modification,(18) to reduce friability and asphalt absorption is encouraged.Bottom ash and boiler slag possess unique physical and engineering properties that are different from conventional pavement materials; therefore, standard test methods may reject bottom ash or boiler slags that would provide acceptable performance. New or modified test methods are needed to characterize bottom ash and boiler slag properties that influence pavement performance. Improved characterization is needed for both abrasion loss and particle size degradation that may occur during compaction.
REFERENCES
A searchable version of the references used in this section is available here. A searchable bibliography of bottom ash and boiler slag literature is available here.- Ksaibati K, Conner GL. Laboratory evaluation of bottom ash asphalt mixes. Department of Civil & Architectural Engineering, University of Wyoming; Laramie, WY: 2004 May.
- Ksaibati K, Sayiri, S. R. K. Utilization of Wyoming bottom ash in asphalt mixes. Department of Civil & Architectural Engineering, University of Wyoming; Laramie, WY: 2006 March.
- NETL National Energy Technology Laboratory. Clean coal technology: Coal utilization by-products. Department of Energy Office of Fossil Energy; Washington, DC: 2006 August. Topical report no. 24.
- American Coal Ash Association (ACAA). 2006 coal combustion product (CCP) production and use. American Coal Ash Association; Aurora, CO: 2007.
- Robnett QL. Use of boiler bottom ash as a paving material A technical database. Georgia Institute of Technology, School of Civil Engineering; Atlanta, GA: 1983.
- Root RE, Williams EG. West Virginia turns waste material into useful aggregate. Asphalt 1976;29(2).
- Usmen M, Anderson DA. Use of power plant aggregate in asphaltic concrete. In: Proceedings of the fourth international ash utilization symposium, report no. MERC/SP-76/4. Washington, DC: U.S. Energy Research and Development Administration; 1976.
- Moulton LK, Seals RK, Anderson DA. Utilization of ash from coal burning power plants in highway construction. Transportation Research Record 1973(430):26-39.
- Kerkhoff GO. Bottom ash and wet bottom slag. In: Presented at the annual soils engineers meeting of the Michigan department of transportation. Lansing, Michigan: Michigan Department of Transportation; 1968.
- Cockrell CF, Shafer HE, Leonard JW. New or undeveloped methods for producing and utilizing coal ash. In: Proceedings of the second international ash utilization symposium, information circular no. 8488. Washington, DC: U. S. Bureau of Mines; 1970.
- American Coal Ash Association (ACAA). Boiler slag. American Coal Ash Association; Aurora, CO: 2004 August. Resource Bulletin 23.
- Huang WH. The use of bottom ash in highway embankment and pavement construction. West Lafayette, IN: Purdue University; 1990. p. 317.
- Groppo J, Robl T. Construction fill sand production from bottom ash at Mill Creek Station. EPA; 2003 December. Case study No. 7.
- ASTM D1073-06 standard specification for fine aggregate for bituminous paving mixtures. In: Annual book of ASTM standards. ASTM; West Conshohocken, Pennsylvania: 2006.
- Moulton LK. Bottom ash and boiler slag. In: Proceedings of the third international ash utilization symposium. U.S. Bureau of Mines; Washington, DC: 1973.
- Özkan Ö, Yüksel I, Muratoglu Ö. Strength properties of concrete incorporating coal bottom ash and granulated blast furnace slag. Waste Management 2007;27(2):161-7.
- Ramme BW, Tharaniyil M. Coal combustion products utilization handbook. We Energies; Milwaukee, WI: 2004.
- Estakhri CK, Saylak D. Sulfur-modified bottom ash as aggregate in hot-mix asphalt concrete: Field demonstration project. Transportation Research Record 2000(1723):57-65.
- ASTM C88-05 standard test method for soundness of aggregates by use of sodium sulfate or magnesium sulfate. In: Annual book of ASTM standards. ASTM; West Conshohocken, Pennsylvania: 2005.
- Asokan P, Saxena M, Asolekar S. Coal combustion residues – environmental implications and recycling potentials. Resources, Conservation and Recycling 2005;43:239-62.
- Anderson DA, Usmen M, Moulton LK. Use of power plant aggregate in bituminous construction. In: Presented at the 55th annual meeting of the transportation research board. Washington, DC: Transportation Research Board; 1976.
- ASTM D1075-07 standard test method for effect of water on compressive strength of compacted bituminous mixtures. In: Annual book of ASTM standards. ASTM; West Conshohocken, Pennsylvania: 2007.
- AASHTO. Guide for design of pavement structures. American Association of State Highway and Transportation Officials; Washington, DC,:1993.
- Churchill EV, Amirkhanian SN. Coal ash utilization in asphalt concrete mixtures. Journal of Materials in Civil Engineering 1999;11:295-301.
- Environmental Protection Agency (EPA), Federal Highway Administration (FHWA). Using coal ash in highway construction - A guide to benefits and impacts. ; 2005. Report nr EPA-530-K-002:ID: 151.
Coal Bottom Ash/Boiler Slag - Granular Base
INTRODUCTION
Coal bottom ash and boiler slag have been used as a granular base material in road and parking lot construction. Bottom ash or boiler slag need to satisfy material specifications for gradation, soundness, and abrasion loss. Bottom ash and boiler slag can be blended with natural aggregate to produce a mixture that meets specifications. Due to higher value uses of boiler slag, bottom ash is used more prevalently as a stabilized or unstabilized base or subbase material.PERFORMANCE RECORD
Bottom ash has been successfully used as granular base since the early 1970's. The American Coal Ash Association reported that 740,000 metric tons (815,000 tons) of bottom ash were used as road base or subbase materials in 2006.(1) The road base or subbase category used by the American Coal Ash Association includes the use of coal bottom ash as an unbound base or in stabilized subbase or stabilized base material. Bottom ash is being studied and used as a granular base in both public(2;3;4;5) and private projects, although private use is not well documented in the literature.MATERIAL PROCESSING REQUIREMENTS
Dewatering (Moisture Control)Bottom ash is typically free-draining, therefore, unless saturated, the moisture content has little influence on compaction characteristics.(6) Short-term stockpiling of bottom ash, typically less than two days, may be required to reduce moisture content. Reclaimed ponded ash may require longer-term stockpiling, up to two weeks, to reduce moisture content to an appropriate level. The moisture content of bottom ash should be high enough to prevent dusting during material handling.
Screening
Bottom ash may meet granular base specifications without processing,(2;7) although the ash may require screening, washing, or blending with conventional aggregate to meet specifications. Oversize or agglomerated popcorn particles may be present in some bottom ash sources and should be removed by screening. Because boiler slag is a poorly-graded material, screening is typically not needed, but blending with conventional aggregate may be required.
Deleterious Materials
Deleterious materials, such as soluble sulfates or coal pyrites, should be removed from bottom ash, boiler slag, or pond ash before use as a granular base. Pyrites should be removed from the coal prior to burning and handled separately from the ash. The pyrites should not be commingled with the ash stream. Although an added cost, processing techniques do exist to remove pyrites from bottom ash.8
ENGINEERING PROPERTIES
Engineering properties of bottom ash and/or boiler slag that are important in granular base applications are gradation, specific gravity, unit weight, compaction characteristics, degradation under compaction, shear strength, bearing strength (CBR), resilient modulus, corrosivity, and hydraulic conductivity.Gradation: Bottom ash and boiler slag are considered fine aggregates in a granular base.(9;10) To improve grain size distribution characteristics of bottom ash or boiler slag, a conventional aggregate or a slag aggregate may be blended with the ash.
Specific Gravity: The specific gravity of bottom ash is in the range of 2.1 to 2.7,(11) but values as low as 1.9 and as high as 3.4 have been recorded.(12) Bottom ash with relatively low apparent specific gravity is often indicative of the presence of porous particles trapping gases that effect the test results. Bottom ash with relatively high specific gravity may indicate a high iron content. The specific gravity of boiler slag is in the range of 2.3 to 2.9.
Dry Unit weight: The dry unit weight of bottom ash is in the range of 7.07 to 15.72 kN/m3 (45 to 100 lb/ft3) while the dry unit weight of boiler slag is in the range of 9.43 to 14.15 kN/m3 (60 to 90 lb/ft3).(11) The dry unit weight of bottom ash can reach as high as 18.08 kN/m3 (115 lb/ft3) while the dry unit weight of boiler slag may be as high as 17.29 kN/m3 (110 lb/ft3).(12)
Compaction Characteristics: The laboratory compaction curves for dry bottom ash are similar in shape to those of typical cohesionless materials. These curves are characterized by a relatively high dry density for the air-dried condition, a lower dry density at intermediate moisture, and a high dry density at or near saturation. Generally, field compaction curves also exhibit maximum dry density at either an air-dried condition or a flushed or wet condition.(12) Although difficult to maintain an air-dried condition in the field, a "flushed" condition can be maintained, which should produce a maximum dry density.(13) When compared to conventional granular materials, bottom ashes have lower maximum dry densities.(14) Bottom ash studied by WE Energies exhibited a maximum dry density of 13.91 kN/m3 (88.5 lb/ft3) and an optimum moisture content of 28 percent.(15) Conventional aggregates have maximum densities in the range of 16.51 to 18.87 kN/m3 (105 to 120 lb/ft3) at optimum moisture content typically in the range of 8 to 16 percent.(15)
Degradation Under Compaction: To quantify the extent of degradation under compaction, an index of crushing has been developed by calculating the mean size of a material before and after compaction and expressing the index of crushing as the percent reduction between the two mean sizes. The higher the index, the easier a material crushes. The index of crushing for coal bottom ash from a pulverized coal boiler was found to be roughly twice that of conventional aggregates, whereas the index of crushing for boiler slag is essentially the same as that of conventional aggregates. The index of crushing for bottom ash from a stoker-fired boiler was found to be about three times greater than the index of crushing for bottom ash from a pulverized coal boiler.(12)
Shear Strength: Bottom ash, having a rough surface texture and angular particles, has a slightly higher friction angle than conventional granular soils when compacted to a high relative density. Direct shear tests conducted on dry bottom ash samples under loose and dense relative density conditions indicated that the angle of internal friction for most bottom ashes ranged from 35 to 50 degrees, depending on the extent of densification, with some dense bottom ash samples exhibiting friction angles as high as 55 degrees. The angle of internal friction for boiler slag was found to fall within the same range as that of most natural granular soils (between 36 and 46 degrees).(11)
Bearing Strength: California Bearing Ratio (CBR) tests on bottom ash indicate that soaking does not negatively affect CBR.(12) Although the maximum CBR is obtained for bottom ash compacted slightly dry of optimum, where optimum is approximately a flushed conditioned, the general trend for CBR values is to increase with increasing moisture contents.(13) The CBR values of bottom ash compacted a high moisture contents were found to be higher (CBR 40 to 70) than the CBR values of bottom ash compacted at low moisture contents (CBR 35 to 60) indicating that compacting at high moisture contents is advantageous.
In general, more coarsely graded and more angular materials tend to exhibit greater stiffness and tend to distribute load more evenly. When the same thickness is used, bottom ash exhibits less load distribution characteristics and would be more flexible than conventional aggregates, (15) even though bottom ash falls in the categories of "good subbases" and "good gravel bases" on the basis of CBR values.(13).
Resilient Modulus: Resilient modulus is a measure of the modulus of elasticity during rapidly applied loadings. Resilient modulus is related to the long-term performance of materials under service loads. Unbound bottom ash tested following AASHTO T 292 protocol produced a resilient modulus of 200 to 400 MN/m2.(2) Resilient modulus is effected by the state of stress, but even when corrections are applied, the laboratory modulus for bottom ash tends to be lower (by a factor of 4) than the modulus calculated from field tests.(3)
Corrosivity: Bottom ash or boiler slag used as a granular base may potentially corrode metal structures.(16) Parameters of interest that are related to corrosivity are pH, electrical resistivity, soluble chlorides, and soluble sulfates. A study of 11 bottom ash or boiler slag samples from Indiana indicated that seven of the samples were considered corrosive, principally because of low electrical resistivity,(16) although pH measurements may exhibit high alkalinity indicating low corrosion potential.(17) Therefore, bottom ash, boiler slag, and ponded ash should be investigated for corrosivity with multiple methods if there is a potential that the ash will come in contact with metal.
Hydraulic Conductivity: The hydraulic conductivity of bottom ash varies between 1 and 10-3 cm/s which is comparable to natural materials with similar grain size distribution.(18) Bottom ash is well draining material and is non-susceptible to frost heave.
The physical properties of coal bottom ash, boiler slag, and ponded ash will vary depending on the type, source, and fineness of the parent fuel, as well as the operating conditions of the power plant;(19) therefore, material specific testing is recommended.
DESIGN CONSIDERATIONS
Pavement design that includes bottom ash, boiler slag, or ponded ash as an unbound or granular base or subbase material can follow AASHTO methods provided in Guide for Design of Pavement Structures.(16) The AASHTO method accounts for the predicted loading (the predicted number of 80 kN equivalent single axle loads), required reliability (degree of certainty that a design will function properly during the design life), serviceable life (ability to maintain quality during the pavement life), the pavement structure (characterized by the structural number), and subgrade support (related to the resilient modulus of the subgrade).(16)The structural number of a pavement design accounts for the relative strength of the constructed materials. The total structural support from the surface course, base course, and any subbase course needs to equal the required structural number. Layer thicknesses are calculated using layer coefficients that define the structural support. The layer coefficients can be obtained from the relationship provided by AASHTO based on CBR or MR.(16)
A layer coefficient value of 0.10 can be used for the design of flexible pavement systems in which bottom ash, boiler slag, or reclaimed ponded ash are used to construct an unbound or granular base or subbase. A coefficient of 0.10 for bottom ash and/or boiler slag recognizes that bottom ash and/or boiler slag are not structurally equivalent to crushed stone, which is typically given a larger coefficient of 0.15.
Bottom ash used at approximately 1.5 times the thickness of conventional aggregates achieves a comparable stress level in the underlying subgrade. For equivalent deformation, the thickness of bottom ash should be two times the thickness of conventional aggregates to maintain similar deflection at the surface of the base course layer.(15)
Compacted unbound bottom ash used as a working platform and subsequently as a contributing subbase member in flexible pavement design has been studied.(2;20;4)Laboratory and case study results show that with proper design and construction, compacted bottom ash provides adequate support as a working platform or subbase material.(2;20) Design charts for selecting the equivalent thickness of compacted bottom ash for working platforms are provided in reference 20, where a methodology for including the structural contribution of working platforms made from bottom ash or other alternative material is presented in 4.
CONSTRUCTION PROCEDURES
Material Handling and StorageBoth bottom ash and boiler slag can be handled and stored using the same methods and equipment that are used for conventional aggregates.
Placing and Compacting
Bottom ash and boiler slag can be dumped and spread with a motor grader or bulldozer or for more accurate grade control, these materials can be placed with a spreader box or paving machine. Bottom ash and boiler slag should be compacted at, or slightly above, optimum moisture content as determined by standard Proctor compaction procedures.(1) Bottom ash loses stability at low moisture contents; therefore, high moisture contents should be maintained to allow construction equipment to operate. The addition of up to 30 percent fines in the form of fly ash may remedy the loss of stability upon drying.(11) Compaction of bottom ash and boiler slag bases and subbases can be accomplished by static steel-wheeled rollers, pneumatic rollers, or vibratory compaction equipment.
After compaction, a bottom ash granular base layer should be protected. A prime coat of asphalt emulsion can be applied to the base material to prevent moisture evaporation, stabilize the surface, and provide a bond between the base layer and an asphalt or Portland cement concrete wearing surface. An asphalt binder, wearing surface, or concrete pavement should be constructed within a reasonable time after sealing a granular base layer to minimize traffic loads on the base layer.
ENVIRONMENTAL CONSIDERATIONS
As described in the Coal Bottom Ash\Boiler Slag Material Description, the use of bottom ash or boiler slag as an unbound granular base is an unencapsulated use and therefore has the potential to leach trace elements. Use of bottom ash or boiler slag as an unbound granular base requires good management and care to ensure that there is no negative impact on the environment. In particular, areas with sandy soils possessing high hydraulic conductivities and areas near shallow groundwater or drinking aquifers should be given careful consideration. An evaluation of groundwater conditions, applicable state test procedures, water quality standards, and proper construction are all necessary considerations in ensuring a safe product that does not adversely affect the environment.(21)UNRESOLVED ISSUES
Bottom ash and boiler slag aggregates possess somewhat unique engineering properties when compared to conventional aggregates. For example, bottom ash may contain some particles that can crush or degrade easily, while boiler slag is very uniformly graded. Some sources of bottom ash or boiler slag, which provide satisfactory performance as an unbound granular base, may not satisfy all specification requirements. This is particularly the case for particle size distribution specifications and abrasion loss requirements for graded base courses. Performance based specifications rather than material characteristic specifications would allow for an increased use of unconventional materials as unbound granular bases.REFERENCES
A searchable version of the references used in this section is available here. A searchable bibliography of bottom ash and boiler slag literature is available here.- American Coal Ash Association (ACAA). 2006 coal combustion product (CCP) production and use. American Coal Ash Association; Aurora, CO: 2007.
- Edil TB, Benson CH, Bin-Shafique MS, Tanyu BF, Kim W, Senol A. Field evaluation of construction alternatives for roadways over soft subgrade. Transportation Research Record 2002;(1786):36-48.
- Tanyu BF, Kim W, Edil TB, Benson CH. Comparison of laboratory resilient modulus with back-calculated elastic moduli from large-scale model experiments and FWD tests on granular materials, In: G. Durham, A. Marr, W. De Groff, editors. Resilient modulus testing for pavement components. West Conshohocken, PA: ASTM; 2003.
- Tanyu BF, Kim W, Edil TB, Benson CH. Development of methodology to include structural contribution of alternative working platforms in pavement structure. Transportation Research Record 2005(1936):70-7.
- Seals RK, Moulton LK, Ruth BE. Bottom ash: An engineering material. Journal of the Soil Mechanics and Foundations Division 1972 April; SM 4:311-25.
- ASTM E2277-03 standard guide for design and construction of coal ash structural fills. In: Annual book of ASTM standards. West Conshohocken, Pennsylvania: American Society for Testing and Materials; 2003.
- Moulton LK, Seals RK, Anderson DA. Utilization of ash from coal burning power plants in highway construction. Transportation Research Record 1973(430):26-39.
- Groppo J, Robl T. Construction fill sand production from bottom ash at mill creek station. EPA; 2003 December. Case study No. 7.
- ASTM. ASTM D1241-07 standard specification for materials for soil-aggregate subbase, base, and surface courses. In: Annual book of ASTM standards. West Conshohocken, Pennsylvania: ASTM; 2007.
- ASTM D2940-03 standard specification for graded aggregate material for bases or subbases for highways or airports. In: Annual book of ASTM standards. West Conshohocken, Pennsylvania: American Society for Testing and Materials; 2003.
- Moulton LK. Bottom ash and boiler slag. In: Proceedings of the third international ash utilization symposium. Washington, DC: U.S. Bureau of Mines; 1973.
- Lovell CW, Ke TC, Huang WH, Lovell JE. Bottom ash as highway material. In: 70th annual meeting of the transportation research board. Transportation Research Board; Washington, DC:1991.
- Huang WH. The use of bottom ash in highway embankment and pavement construction. Purdue University; West Lafayette, IN: 1990. p. 317
- Usmen MA. A critical review of the applicability of conventional test methods and materials specifications to the use of coal-associated wastes in pavement construction.: West Virginia University; Morgantown, West Virginia: 1977.
- Ramme BW, Tharaniyil M. Coal combustion products utilization handbook. We Energies; Milwaukee, WI: 2004.
- AASHTO. Guide for design of pavement structures. American Association of State Highway and Transportation Officials; Washington, DC,: 1993.
- Kim B, Prezzi M. Compaction characteristics and corrosivity of indiana class-F fly and bottom ash mixtures. Construction and Building Materials; In Press, 2007.
- Rogbeck J, Knutz A. Coal bottom ash as light fill material in construction. Waste Management 1996;16(1-3):125-8.
- Özkan Ö, Yüksel I, Muratoglu Ö. Strength properties of concrete incorporating coal bottom ash and granulated blast furnace slag. Waste Management 2007;27(2):161-7.
- Tanyu BF, Benson CH, Edil TB, Kim W. Equivalency of crushed rock and three industrial by-products used for working platforms during pavement construction. Transportation Research Record 2004(1874):59-69.
- Environmental Protection Agency (EPA), Federal Highway Administration (FHWA). Using coal ash in highway construction - A guide to benefits and impacts. ; 2005. Report nr EPA-530-K-002:ID: 151.
Coal Bottom Ash/Boiler Slag - Stabilized Base
INTRODUCTION
Bottom ash or boiler slag can be used as the fine aggregate fraction or as the entire aggregate in Portland cement or pozzolan-stabilized base and subbase mixtures. Bottom ash and, in particular, boiler slag have been used as aggregate in stabilized base or subbase applications since the 1950's. Most installations have not been well documented, but there is no indication of unsatisfactory performance.PERFORMANCE RECORD
In 2006, the American Coal Ash Association reported that over 740,000 metric tons (815,000 tons) of bottom ash were used as road base or subbase materials.(1) The road base or subbase category used by the American Coal Ash Association includes the use of coal bottom ash as an unbound base, stabilized subbase, or stabilized base material.Pozzolan-stabilized base compositions consisting of lime, fly ash, and aggregates (LFA) were originally patented in the early 1950's under the trade name Poz-O-Pac. Some of the first LFA compositions were mixed in place and used boiler slag as the aggregate. These early mixtures contained an average of 5 percent by weight hydrated lime, 35 percent Class F fly ash, and 60 percent boiler slag. Pavements using such mixtures provided many years of service and cores taken from these pavements have developed compressive strengths over 6900 kPa (1000 lb/in2).
Case studies report that fly ash stabilized base courses may contain 65 percent bottom ash or boiler slag by weight, while Portland cement mixes may contain up to 95 percent bottom ash or boiler slag. The remaining percentage of a pozzolan stabilized base mix is fly ash, lime, or Portland cement. Depending on the blending with natural aggregates, bituminous stabilized bottom ash or boiler slag base courses require between 5 to 7 percent asphalt content.(2)
MATERIAL PROCESSING REQUIREMENTS
Bottom ash and boiler slag are both well-draining materials that can be dewatered in 1 or 2 days. Ponded ash, which typically contains fly ash, requires a longer time to dewater, up to two weeks. The higher the percentage of fly ash in ponded ash, the longer the dewatering time.Crushing or Screening
Bottom ash is generally a more well-graded aggregate than boiler slag, which is normally more uniformly graded between the No. 4 (4.75 mm) and No. 40 (0.42 mm) sieves. Pond ash may be a blend of bottom ash and fly ash, and will vary in gradation, depending on the proximity to the discharge pipe in a lagoon. Bottom ash may contain some agglomerations or popcorn-like particles. These agglomerations should either be reduced in size by clinker grinders at the power plant or removed by scalping or screening at the 12.7 mm (½ in) or 19 mm (¾ in) screen.
Blending
As produced, bottom ash may meet gradation specifications for stabilized base or may require blending with other coal combustion products or natural aggregates to meet specifications. Boiler slag, being poorly-graded, will require blending to meet gradation specifications. Well-graded aggregates normally require less activator or reagent than poorly graded aggregates in order to produce a well-compacted stabilized base.
Removal of Deleterious Materials
Deleterious materials in bottom ash or boiler slag, especially coal pyrites, should be removed prior to use as an aggregate. Pyrites oxidize (or weather) over time, causing expansion and popouts of individual particles from the matrix. Soluble sulfates exist in some bottom ashes. Low pH values in bottom ash are often used as an indicator for the presence of sulfates. Technologies exist for processing bottom ash that can provide a cost-effective method to remove impurities (i.e. unburnt coal and pyrite) so that bottom ash meets product quality targets.(3)
ENGINEERING PROPERTIES
Engineering properties of bottom ash and boiler slag that are important when using this material as aggregate in stabilized base or subbase mixtures are gradation, specific gravity, unit weight, durability, and soundness.Gradation: The size limits in Table 4 are recommended for cement-treated aggregate base by the Portland Cement Association and are applicable to bottom ash or boiler slag use in cement-treated base course mixes.(4)
Sieve Size | Percent Passing |
19 mm (3/4 in) | 100 |
9.5 mm (3/8 in) | 70-90 |
4.75 mm (No. 4) | 55-90 |
3.35 mm (No. 8) | 40-70 |
1.18 mm (No. 16) | 30-60 |
0.075 mm (No. 200) | 0-30 |
Specific Gravity: The specific gravity of bottom ash is in the range of 2.1 to 2.7,(5) but values as low as 1.9 and as high as 3.4 have been recorded.(6) Bottom ash with relatively low apparent specific gravity is often indicative of the presence of porous particles trapping gases that effect the test results. Bottom ash with relatively high specific gravity may indicate a high iron content. The specific gravity of boiler slag is in the range of 2.3 to 2.9.
Dry Unit weight: The dry unit weight of bottom ash is in the range of 7.07 to 15.72 kN/m3 (45 to 100 lb/ft3) while the dry unit weight of boiler slag is in the range of 9.43 to 14.15 kN/m3 (60 to 90 lb/ft3).(5) The dry unit weight of bottom ash can reach as high as 18.08 kN/m3 (115 lb/ft3) while the dry unit weight of boiler slag may be as high as 17.29 kN/m3 (110 lb/ft3).(6)
Durability: Bottom ash and boiler slag exhibit marginal durability as measured by ASTM C131 (Los Angeles Abrasion) tests, with bottom ash percent loss values between 30 and 50 and boiler slag between 24 and 48 percent.(5) Most bottom ash samples have some friable particles, while boiler slag normally does not. Los Angeles Abrasion test results have shown that bottom ash samples are not as sound or durable as natural aggregate. However, the test results fall within the specifications of a maximum 50 percent loss by abrasion.(7)
Soundness: The durability of an aggregate used in stabilized bases or subbases can be evaluated by the sodium sulfate soundness test. Bottom ash has had sodium sulfate soundness loss values that normally range from 1.5 to 10.5 percent.(5) Boiler slag has had sodium sulfate soundness loss values of between 1 and 9 percent.(5) Lower the specific gravity may indicate a higher percentage of deleterious material, which will be reflected in a higher soundness loss.
DESIGN CONSIDERATIONS
Mix DesignFor pozzolan-stabilized base (PSB) mixtures made with bottom ash or boiler slag and containing coal fly ash (along with lime, Portland cement, or kiln dust as an activator), the initial step in determining mix design proportions is to find the optimum fines content. This is done by progressively increasing the percentage of fines and determining the compacted density of each blend. Each blend of aggregate and fines is compacted into a Proctor mold using standard compaction procedures. Fly ash percentages ranging from 25 to 45 percent by dry weight of the total blend are suggested for the initial trial mixes. The optimum fines content selected by this procedure should be 2 percent higher than the fines content at the maximum dry density. The optimum moisture content must then be determined for this mix design.
Once the design fly ash percentage and optimum moisture content have been determined, the ratio of activator to fly ash must be determined. Using a series of trial mixtures, final mix proportions are selected on the basis of the results of both strength and durability testing according to ASTM C593 procedures.(8)
For cement-stabilized bottom ash and boiler slag mixtures, the only mix design consideration is the percentage of Portland cement. Trial mixes between 5 and 12 percent Portland cement are needed to properly stabilize bottom ash or boiler slag for use as a roller-compacted base course. The results of ASTM C593 compressive strength and durability testing should be the basis for selection of the final mix.
The compacted unit weight of bottom ash or boiler slag mixes is lower than the compacted unit weight of stabilized base mixtures containing conventional aggregates. Consequently, a cement content of 10 percent by weight for a base course mix containing bottom ash or boiler slag may be equivalent to 7 percent by weight cement content for a similar mix containing a natural aggregate.
The trial mixture with the lowest percentage of cement (or activator plus fly ash in PSB mixtures) that satisfies both the compressive strength and the durability criteria is considered the most economical mixture. To ensure an adequate factor of safety for field placement, stabilized base or subbase mixture used in the field should have an activator content that is at least 0.5 percent higher (1.0 percent higher if using kiln dust) than that of the most economical mixture.(9)
Structural Design
Designing pavement structures that include stabilized base layers with bottom ash or boiler slag aggregate should follow AASHTO pavement design methods provided in the Guide for Design of Pavement Structures,(10) or the Guide for the Mechanistic-Empirical Design of New and Rehabilitated Pavement Structures.(11) The AASHTO methods account for the predicted loading (the number of 80 kN equivalent single axle loads), required reliability (degree of certainty that a design will function properly during the design life), serviceable life (ability to maintain quality during the pavement life), the pavement structure (characterized by the structural number), and subgrade support (related to the resilient modulus of the subgrade).(10)
A hierarchical approach in the mechanistic-empirical design method allows for varying levels of material characterization depending on project criteria. Mechanistic material properties such as dynamic modulus, resilient modulus, and Poisson’s ratio are employed to evaluate pavement performance. The levels in the hierarchical system can directly measure strength characteristics (level 1), can use correlations to develop strength characteristics (level 2), or can use typical material property default values (level 3). Both asphalt stabilized base materials and chemically or cement stabilized base materials are included in the mechanistic-empirical design method under different material categories.
Pavement design employing a structural number accounts for the relative strength of the constructed materials. The total structural support from the surface course, base course, and any subbase course equals the required structural number. Layer thicknesses are calculated using layer coefficients that define the structural support. The layer coefficients can be obtained from the relationship provided by AASHTO based on CBR or MR.(10)
When a Portland cement concrete roadway surface is to be designed with a stabilized base or subbase, the AASHTO structural design method for rigid pavements can be used.(10)
CONSTRUCTION PROCEDURES
Material Handling and StorageBoth bottom ash and boiler slag can be handled and stored using methods and equipment that are used for conventional aggregates.
Mixing, Placing, and Compacting
The blending or mixing of bottom ash or boiler slag in stabilized base mixtures can be done in a mixing plant or in place. Plant mixing provides control over the quantities of materials batched, resulting in a uniform mixture. Mix proportions from in-place mixing are not as accurate as mix proportions from plant mixing, although in-place mixing of mixes containing bottom ash or boiler slag produce satisfactory stabilized base material.
Stabilized base materials should not be placed in layers that are less than 100 mm (4 in) or greater than 200 to 225 mm (8 to 9 in) in compacted thickness. Stabilized base material should be spread in loose layers that are approximately 50 mm (2 in) thicker than the compacted thickness. The top surface of an underlying layer should be scarified prior to placing the next layer. For granular or coarse graded mixtures, steel-wheeled vibratory rollers are used. For fine-grained mixtures, a vibratory sheepsfoot roller, followed by a pneumatic roller, provide quality stabilized base compaction.(9)
To develop the design strength of a stabilized base mixture, the material should be well-compacted at the optimum moisture content. To avoid drying, plant-mixed materials should be delivered to the job site as soon as possible and should be compacted within a reasonable time after placement.
Compaction of fly ash stabilized bottom ash or boiler slag mixtures should be completed as quickly as possible after placement. The stabilized material can lose strength capacity if the fly ash hydrates in an uncompacted state. The pozzolanic reaction between Class F fly ash and lime is a relatively slow reaction, and a maximum delay of 4-hours should be followed whereas a maximum delay of 2-hours is recommended for Class C fly ash.(12;13) To slow the reaction, a commercial retarder, such as gypsum or borax, can be added at the mixing plant in low percentages (approximately 1 percent by weight) without adversely affecting the strength development of the stabilized base material.(9)
Curing
After placement and compaction, the stabilized base material should be properly cured to protect against drying and to assist in the development of in-place strength. An asphalt emulsion seal coat should be applied within 24 hours after placement. The same practice is applicable if a Portland cement concrete or asphalt pavement is constructed above the stabilized base or subbase material. Paving over the stabilized base is recommended within 7 days after the base has been installed. Unless an asphalt binder and/or surface course has been placed over the stabilized material, vehicles should not drive over the stabilized base until an in-place compressive strength of at least 2400 kPa (350 lb/in2) has been achieved.(9)
SPECIAL CONSIDERATIONS
Cold Weather Construction: Stabilized base materials containing bottom ash or boiler slag that are subjected to freezing and thawing conditions should develop a level of in-place strength prior to the first freeze-thaw cycle. For northern states, many state transportation agencies have established construction cutoff dates for stabilized base materials. These cutoff dates range from September 15 to October 15, depending on the state, or the location within a particular state.(10)Crack Control Techniques: Stabilized base materials, especially those in which Portland cement is used as the activator, are subject to cracking. The cracks are typically shrinkage related and are not the result of structural weakness or defects in the stabilized base material. The cracks also not related to the type of aggregate used in the mix. Shrinkage cracks will reflect through the overlying asphalt pavement and should be sealed at the pavement surface to prevent water intrusion and subsequent damage due to freezing and thawing.
An approach to controlling or minimizing reflective cracking associated with shrinkage cracks in stabilized base materials is to saw cut transverse joints in the asphalt surface that extend into the stabilized base material to a depth of 75 to 100 mm (3 to 4 in). Joint spacing of 9 m (30 ft) have been suggested.(9) Joints should be sealed with a material such as hot poured asphaltic joint sealant.
ENVIRONMENTAL CONSIDERATIONS
Although stabilized, bottom ash and boiler slag used in stabilized base and subbase layers is considered an unencapsulated use due to the high hydraulic conductivity. Therefore, bottom ash and boiler slag have the potential to leach trace elements. Use of bottom ash and boiler slag in base material requires good management and care to ensure that the material does not result in a negative impact on the environment. In particular, areas with sandy soils possessing high hydraulic conductivities and areas near shallow groundwater or drinking aquifers should be given careful consideration. An evaluation of groundwater conditions, applicable state test procedures, water quality standards, and proper construction are all necessary considerations in ensuring a safe final product.(14)UNRESOLVED ISSUES
As noted above, control of shrinkage cracking has been long considered by many state transportation agencies as a prime concern associated with stabilized base mixtures, especially cement-stabilized mixtures. Since most mixtures that include bottom ash or boiler slag as the aggregate have been placed on secondary roads, haul roads, and parking lots, as opposed to higher-type highway facilities, the issue of crack control has not been a major concern. However, additional mix designs with reduced potential for shrinkage cracking need to be developed, especially when these materials are used on higher-type facilities.Pyrites should be removed before bottom ash or boiler slag is used as aggregate in a stabilized base. Soluble sulfates in bottom ash may warrant removal if found in sufficient quantity to be considered detrimental. Improved techniques for economic removal of these constituents are needed.
REFERENCES
A searchable version of the references used in this section is available here. A searchable bibliography of bottom ash and boiler slag literature is available here.- American Coal Ash Association (ACAA). 2006 coal combustion product (CCP) production and use. American Coal Ash Association; Aurora, CO: 2007.
- Moulton LK, Seals RK, Anderson DA. Utilization of ash from coal burning power plants in highway construction. Transportation Research Record 1973(430):26-39.
- Groppo J, Robl T. Construction fill sand production from bottom ash at Mill Creek Station. EPA; 2003 December. Case study No. 7.
- Halsted GE, Luhr DR, Adaska WS. Guide to cement-treated base (CTB).: Portland Cement Association; Skokie, IL 2007.
- Moulton LK. Bottom ash and boiler slag. In: Proceedings of the third international ash utilization symposium. U.S. Bureau of Mines; Washington, DC: 1973. 6. Lovell CW, Ke TC, Huang WH, Lovell JE. Bottom ash as highway material. In: 70th annual meeting of the transportation research board. Washington, DC: Transportation Research Board; 1991.
- Ramme BW, Tharaniyil M. Coal combustion products utilization handbook. We Energies; Milwaukee, WI: 2004.
- ASTM C593-06 standard specification for fly ash and other pozzolans for use with lime for soil stabilization. In: Annual book of ASTM standards. ASTM; West Conshohocken, Pennsylvania: 2006.
- American Coal Ash Association (ACAA). Flexible pavement manual: Recommended practice - coal fly ash in pozzolanic stabilized mixtures for flexible pavement systems. 1991:128 p.
- AASHTO. Guide for design of pavement structures. American Association of State Highway and Transportation Officials; Washington, DC: 1993.
- NCHRP 1-37A. Guide for mechanistic – empirical design of new and rehabilitated pavement structures. National Cooperative Highway Research Program, Transportation Research Board; 2004.
- White DJ, Harrington D, Thomas Z. Fly ash soil stabilization for non-uniform subgrade soils, volume I: Engineering properties and construction guidelines. Iowa State University, Ames, IA: Center for Transportation Research and Education Iowa State University; 2005.
- Little DN, Males EH, Prusinski JR, Stewart B. Cementitious stabilization. In: Transportation in the new millennium: State of the art and future directions, perspectives from TRB standing committees. Transportation Research Board, National Research Council; Washington, DC: 2000.
- Environmental Protection Agency (EPA), Federal Highway Administration (FHWA). Using coal ash in highway construction - A guide to benefits and impacts. ; 2005. Report nr EPA-530-K-002:ID: 151.
Coal Bottom Ash/Boiler Slag - Embankment/Structure Fill
INTRODUCTION
According to the American Coal Ash Association approximately 3.63 million metric tons (4.0 million tons) of bottom ash were utilized in structural fill and embankment applications in 2006.(1) Structural fill and embankment material is the largest use of bottom ash in the U.S. While specifications for bottom ash and boiler slag reuse depend on the application, there are material characteristics that must be met when using bottom ash or boiler slag in embankments or as a structural fill.PERFORMANCE RECORD
Bottom ash and boiler slag have been successfully used as embankment or structural fill material both nationally and internationally. Bottom ash from WE Energies of Wisconsin was successfully used as a backfill material in Racine, Wisconsin(2) as well as fill for a freeway spur in Milwaukee, Wisconsin and other projects.(3) Indiana DoT has used bottom ash in numerous transportation applications including as an embankment material.(4) Approximately 1 million metric tons (1.1 million tons) of coal combustion products (mixtures of fly ash, bottom ash, boiler slag, and fluidized bed combustion ash) were used to raise embankments on an ash pond in Rihand, India.(5) Coal combustion products including bottom ash have also been used in India for widening existing bridge approaches or building embankments for overpass bridges in Delhi.(6)Bottom ash has been used as a light weight fill over soft soils in Sweden due to the low unit weight of bottom ash.(7)ENGINEERING PROPERTIES
Mechanical characteristics of bottom ash that are important when bottom ash is used as an embankment or fill material are: moisture-density relationship (compaction curve), hydraulic conductivity, particle distribution, strength, stiffness, and compressibility.Moisture-Density Relationship: Bottom ash and boiler slag are coarse-grained materials that are relatively insensitive to moisture content during compaction.(8) Compacted dry unit weight of bottom ash is in the range of 15.7 to 17.6 kN/m3 (100 to 112 lb/ft3), but can be as low as 9.9 kN/m3 (63 lb/ft3).(9) Dry bottom ash can sustain particle degradation during compaction.(9) Crushing of the bottom ash particles during compaction contributes to an increase in the maximum dry unit weight. Compaction characteristics of mixtures of fly ash and bottom ash have more well-graded size distributions, which allows the fly and bottom ash particles to pack more closely, resulting in an increase in the maximum dry unit weight of the mixture.(10)
Hydraulic Conductivity: The hydraulic conductivity of well-compacted bottom ash ranges from 1 to 10-3 cm/s, which is roughly equivalent to the hydraulic conductivity of sand.(7) Bottom ash is free draining and not typically susceptible to either liquefaction or frost heave.(11)
Particle Size Distribution: Bottom ash is a well-graded material with particles ranging in size from fine gravel to fine sand with low percentages of silt-clay sized particles. Bottom ash is predominantly sand-sized, usually with 50 to 90 percent passing the No. 4 sieve (4.75 mm) and 0 to 10 percent passing the No. 200 sieve (0.075 mm). Top particle size for bottom ash is typically between 19 mm (¾ in) to 38.1 mm (1-½ in).
Shear Strength: Bottom ash, having a rough surface texture and angular particles, has a slightly higher friction angle than conventional granular soils. Direct shear tests conducted on bottom ash samples under loose and dense relative density conditions indicated that the angle of internal friction for most bottom ashes ranged from 35 to 50 degrees, depending on the extent of densification, with some dense bottom ash samples exhibiting friction angles as high as 55 degrees. The angle of internal friction for boiler slag was found to fall within the same range as that of most natural granular soils (between 36 and 46 degrees).(12)
Bearing Strength: California Bearing Ratio (CBR) tests on bottom ash indicate that soaking does not negatively affect CBR.(13) Although the maximum CBR is obtained for bottom ash compacted slightly dry of optimum, where optimum is approximately a flushed conditioned, the general trend for CBR values is to increase with increasing moisture contents.(9) The CBR values of bottom ash compacted a high moisture contents were found to be higher (CBR 40 to 70) than the CBR values of bottom ash compacted at low moisture contents (CBR 35 to 60) indicating that compacting at high moisture contents is advantageous.
Compressibility: Granular materials with angular particles are typically more compressible than those with well-rounded particles because the sharp edges of the angular particles tend to be break during compression as well as shear. Although some bottom ash particles are porous and weak, for low stress levels, the compressibility of bottom ash is comparable to that of sand placed at the same relative density.(14) Coal ash consolidates rapidly, therefore compressibility typically is not a design concern.(11)
Table 2 in the Material Description section for Coal Bottom Ash\Boiler Slag lists typical values for engineering parameters of interest when designing with bottom ash or boiler slag.
CONSTRUCTION PROCEDURES
Material Handling and StorageBoth bottom ash and boiler slag can be handled and stored using the same methods and equipment that are used for conventional aggregates.
Placing and Compacting
Prior to placement, a site should undergo preparations consistent with preparation requirements for soil fill materials. Construction equipment needed to properly place and compact bottom ash or boiler slag in an embankment or structural backfill includes a bulldozer for spreading the material, a compactor, either a vibrating or pneumatic tired roller, a water truck to provide water for compaction and to control dusting, and a motor grader where final grade control is critical.
Bottom ash and boiler slag is typically dumped and spread with a bulldozer or motor grader in lifts no thicker that 0.3 m (12 in.) when loose. Bottom ash and boiler slag should be compacted at, or slightly above, optimum moisture content as determined by standard Proctor compaction procedures.(1) Bottom ash loses stability at low moisture contents; therefore, high moisture contents should be maintained to allow construction equipment to operate. The addition of up to 30 percent fines in the form of fly ash may remedy the loss of stability upon drying.(12) Compaction of bottom ash and boiler slag can be accomplished by static steel-wheeled rollers, pneumatic rollers, or vibratory compaction equipment. For each project, the type of compactor, the moisture content of the bottom ash or boiler slag at placement, the lift thickness, and the number of passes of the compaction equipment should be evaluated using a test strip.
Quality Control
Quality control programs for bottom ash or boiler slag embankments or structural backfills are similar to such programs for conventional earthwork projects. These programs typically include visual observations of lift thickness, number of compactor passes per lift, and behavior of fly ash under the weight of the compaction equipment, supplemented by laboratory and field testing to confirm that the compacted material has been constructed in accordance with design specifications.(11) More information on performance specifications and procedures and method specifications and procedures can be found in ASTM E2277.(11)
ENVIRONMENTAL CONSIDERATIONS
As described in the Coal Bottom Ash\Boiler Slag Material Description, the use of bottom ash or boiler slag as an embankment of fill material is an unencapsulated use and therefore has the potential to leach trace elements. Use of bottom ash or boiler slag in embankments or as a fill requires good management and care to ensure that there is no negative impact on the environment. In particular, areas with sandy soils possessing high hydraulic conductivities and areas near shallow groundwater or drinking aquifers should be given careful consideration. An evaluation of groundwater conditions, applicable state test procedures, water quality standards, and proper construction are all necessary considerations in ensuring a safe product that does not adversely affect the environment.(15)REFERENCES
A searchable version of the references used in this section is available here. A searchable bibliography of bottom ash and boiler slag literature is available here.- American Coal Ash Association (ACAA). 2006 coal combustion product (CCP) production and use. Aurora, CO: American Coal Ash Association; 2007. 2. Ramme BW, Tharaniyil M. Coal combustion products utilization handbook. We Energies; Milwaukee, WI: 2004.
- Wisconsin Transportation Information Center. Using recovered materials in highway construction. University of Wisconsin-Madison Department of Engineering Professional Development; Madison, WI: 1999. Wisconsin Transportation Bulletin No. 20.
- Siddiki NZ, Kim D, Salgado R. Use of recycled and waste materials in Indiana. Transportation Research Record 2004(1874):78-85.
- Asokan P, Saxena M, Asolekar S. Coal combustion residues – environmental implications and recycling potentials. Resources, Conservation and Recycling 2005;43:239-62.
- ASTM C331-05 standard specification for lightweight aggregates for concrete masonry units. In: Annual book of ASTM standards. West Conshohocken, Pennsylvania: ASTM; 2005.
- Rogbeck J, Knutz A. Coal bottom ash as light fill material in construction. Waste Management 1996;16(1-3):125-8.
- Edil TB, Benson CH, Bin-Shafique MS, Tanyu BF, Kim W, Senol A. Field evaluation of construction alternatives for roadways over soft subgrade. Transportation Research Record 2002(1786):36-48.
- Huang WH. The use of bottom ash in highway embankment and pavement construction. Purdue University; West Lafayette, IN:1990. p. 317 p.
- Kim B, Prezzi M, Salgado R. Geotechnical properties of fly and bottom ash mixtures for use in highway embankments. Journal of Geotechnical and Geoenvironmental Engineering 2005;131(7):914-24.
- ASTM E2277-03 standard guide for design and construction of coal ash structural fills. In: Annual book of ASTM standards. American Society for Testing and Materials; West Conshohocken, Pennsylvania: 2003.
- Moulton LK. Bottom ash and boiler slag. In: Proceedings of the third international ash utilization symposium. U.S. Bureau of Mines; Washington, DC: 1973.
- Lovell CW, Ke TC, Huang WH, Lovell JE. Bottom ash as highway material. In: 70th annual meeting of the transportation research board. Transportation Research Board; Washington, DC: 1991.
- Seals RK, Moulton LK, Ruth BE. Bottom ash: An engineering material. Journal of the Soil Mechanics and Foundations Division 1972 April; SM 4:311-25.
- Environmental Protection Agency (EPA), Federal Highway Administration (FHWA). Using coal ash in highway construction - A guide to benefits and impacts. 2005. Report nr EPA-530-K-002:ID: 151.